Utilizing Terrestrial Laser Scanning for Estimating Commercial Teak Volume: A Case Study of Mae Chang Forest Plantation, Mae Mo District, Lampang Province
DOI:
https://doi.org/10.34044/tferj.2025.9.1.6085Keywords:
LiDAR, merchantable volume, stem curve, Teak plantationAbstract
Background and Objectives: Teak (Tectona grandis) is one of Thailand's most valuable economic tree species, prized for its exceptional qualities such as durability, aesthetic appeal, and resistance to pests and diseases. Its popularity spans both domestic and international markets, making accurate estimation of merchantable teak volume essential for forest management and the timber industry. Precise volume estimation facilitates accurate economic valuation and minimizes resource losses associated with traditional destructive tree-felling methods. Recent advancements in technology, light detection and ranging or LiDAR, have provided opportunities to enhance the accuracy and efficiency of forest resource data collection. Among these tools, the Terrestrial Laser Scanner (TLS) stands out for its ability to generate high-resolution three-dimensional (3D) models of individual trees and forest stands in a non-destructive method. Unlike traditional methods, TLS enables data collection without damaging trees, offering a sustainable and efficient alternative for forestry applications. This study aimed to develop a methodology for utilizing TLS to construct 3D models of teak trees to estimate merchantable wood volume. The results were compared with the traditional felling method, which is considered the most accurate but destructive. In addition, the study attempts to establish predictive equations and volume tables for estimating merchantable teak volume. These outputs aimed to support sustainable forest management practices, reduce resource wastes, and facilitate the economic valuations of teak for the timber industry.
Methods: The study was conducted in the Mae Chang forest plantation, located in Mae Mo district, Lampang province, Thailand. The stratified random sampling was employed to establish 12 sample plots, each sizing 40 m × 40 m. The plots were divided into two age groups based on plantation year: 18 years old (planted in 2006) and 39 years old (planted in 1985), with six plots in each group. Data collection involved using a Faro Focus S150 TLS to scan individual trees in each plot. The TLS data were processed to create detailed 3D tree models for further analysis. Merchantable timber volume was calculated using two methods: the stem curve method and quantitative structure models (QSM), both implemented through the 3D forest software. The TLS-derived volume estimates were validated by felling selected sample trees. The diameters and merchantable lengths of these felled trees were measured, and their volumes were calculated using Huber’s formula. Statistical analysis was performed to compare TLS-derived volumes with traditional felling-based volumes using paired t-tests. The accuracy of TLS-derived estimates was evaluated using root mean square error (RMSE). Additionally, predictive equations for merchantable volume estimation were developed using regression analysis. Two types of equations were created: one using diameter at breast height (DBH) as the sole variable and the other incorporating both DBH and merchantable height (HM). A volume table was constructed based on these equations, enabling non-destructive volume estimation for teak trees of varying DBH and HM ranges.
Results: The 3D models generated using TLS provided merchantable volume estimates that closely aligned with volumes derived from felled trees. Statistical analysis revealed no significant differences between TLS-derived volumes and traditional felling-based volumes (p > 0.05). The RMSE values for TLS-derived estimates were 0.02 and 0.03 cubic meters when using the stem curve and QSM methods, respectively, indicating high accuracy. Two predictive equations for merchantable volume estimation were developed. The first equation utilized DBH as a single predictor variable and demonstrated a coefficient of determination (R²) of 0.966. The second equation incorporated both DBH and HM, achieving a higher R² value of 0.988. Validation of these equations using data from felled trees showed mean absolute percentage errors (MAPE) of 17.81% and 11.01%, respectively, confirming their suitability for practical applications. The most accurate equation was subsequently used to construct a merchantable volume table, which estimating based on DBH and HM ranges without requiring tree felling. This table provides a practical tool for forest managers and timber industry stakeholders to assess teak volume efficiently and sustainably.
Conclusion: The results of this study demonstrate that TLS is a reliable and effective tool for non-destructive estimation of merchantable teak volume. The 3D models generated using TLS data enable precise volume estimation through methods such as stem curve and QSM, producing results that are statistically comparable to traditional destructive felling methods. The acceptable error margins and high accuracy of TLS-derived estimates highlight its potential as a sustainable alternative to conventional volume estimation techniques. By minimizing the need for destructive sampling, TLS-based methods contribute to sustainable forest management practices, preserving valuable tree resources while maintaining economic productivity. Furthermore, the development of predictive equations and merchantable volume tables offers practical solutions for forest managers and timber industry professionals, streamlining the assessment of merchantable volume and economic valuation. Overall, this study underscores the importance of integrating advanced technologies such as TLS into forestry practices. By improving the accuracy and sustainability of resource assessments, these innovations can support long-term forest conservation and enhance the economic potential of Thailand's teak plantations.
Downloads
References
An, Z. & R. E. Froese. 2022. Tree stem volume estimation from terrestrial LiDAR point cloud by unwrapping. Canadian Journal of Forest Research 53(2): 60-70. doi:10.1139/cjfr-2022-0153
Bornand, A., N. Rehush, F. Morsdorf, E. Thurig & M. Abegg. 2023. Individual tree volume estimation with terrestrial laser scanning: Evaluating reconstructive and allometric approaches. Agricultural and Forest Meteorology 341: 109654. DOI:10.1016/j.agrformet.2023.109654
Buranachan, A. 2022. Yield assessment of trees to support business collateral: a case study of teak in Kroeng Krawia Forest Plantation, Kanchanaburi Province. pp.596-603. In Proceedings of 60th Kasetsart University Annual Conference. Prachacheun Research Network, Pathum Thani. (in Thai)
FARO Technologies Inc. 2019. SCENE 2019 FARO Focus Laser Scanners Training Workbook. USA: Technology Park Lake Mary.
Forest Industry Organizations. 2017. Table of determined teak wood prices from forest plantations by the Forest Industry Organization (FIO). Available source: http://www.fio.co.th/fiopage/doc/price_of_teak_03.pdf, (Accessed: September 17, 2024). (in Thai)
Forest Industry Organizations. 2019. Mae Jang forest plantation management summary. Available source: http://www.northfio.com/web/information/Maejang64.pdf, (Accessed: March 16, 2025). (in Thai)
Hackenberg, J., M. Wassenberg, H. Spiecker & D. Sun. 2015. Non-Destructive Method for Biomass Prediction Combining TLS Derived Tree Volume and Wood Density. Forests 6: 1274-1300. DOI:10.3390/f6041274
Himmapan, W. 2024. Table of total stem volume and merchantable volume of teak trees. Available source: https://online.anyflip.com/unoq/qzwg/mobile/index.html. (Accessed: December 2, 2024) (in Thai)
Husch, B., C. Miller & T. Beers. 1982. Forest mensuration. 3rd ed. John Willey and Sons, New York.
Krooks, A., S. Kaasalainen, V. Kankare, M. Joensuu, P. Raumonen & M. Kaasalainen. 2014. Predicting tree structure from tree height using terrestrial laser scanning and quantitative structure models. Silva Fennica 48: 1-11. DOI:10.14214/sf.1125
Lewis, C. 1982. Industrial and business forecasting methods. Butterworth Scientific, London, UK.
Liang, X., V. Kankare, J. Hyyppä, Y. Wang, A. Kukko, H. Haggrén & M. Vastaranta. 2016. Terrestrial laser scanning in forest inventories. ISPRS Journal of Photogrammetry and Remote Sensing 115: 63-77.
Liu, G., J. Wang, P. Dong, Y. Chen & Z. Liu. 2018. Estimating individual tree height and diameter at breast height (DBH) from terrestrial laser scanning (TLS) Data at Plot Level. Forests 9: 398. DOI:10.3390/f9070398
Panagiotidis, D. & A. Abdollahnejad. 2021. Reliable estimates of merchantable timber volume from terrestrial laser scanning. Remote Sensing 13(18): 3610. DOI: 10.3390/rs13183610
Petrov, M. K., J. Trochta & K. Kral. 2020. 3D Forest user guide. The Silva Tarouca Research Institute, Pub. Res. Inst., Department of Forest Ecology.
Rianthakool, L., J. Yingdee, T. Chumsangsri, C.N. Takuathung, P. Diloksumpun, T. Lakkanasri, C. Tosophon & N. Intim. 2024. The Study of 3D Modelling of Peltophorum pterocarpum with terrestrial laser scanner. Thai Science and Technology Journal 32: 79-91. (in Thai)
Royal Forest Department. 2013. Thai teak knowledge. Bangkok: Ministry of Natural Resources and Environment. (In Thai)
Trochta, J., M. Krucek, T. Vrska & K. Kral. 2017. 3D Forest: An application for descriptions of three-dimensional forest structures using terrestrial LiDAR. PLoS One 12(5): e0176871. DOI:10.1371/journal.pone.0176871
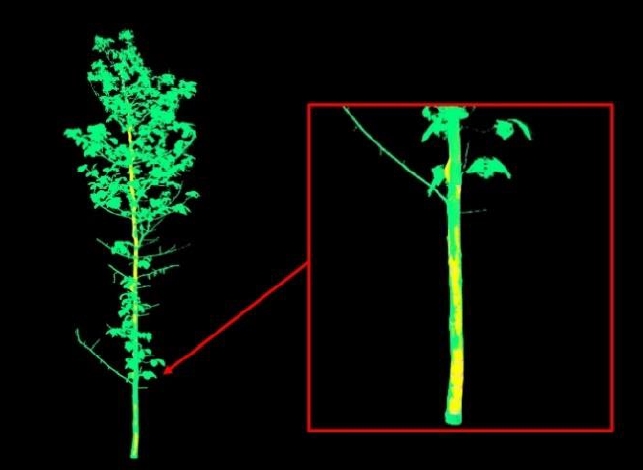